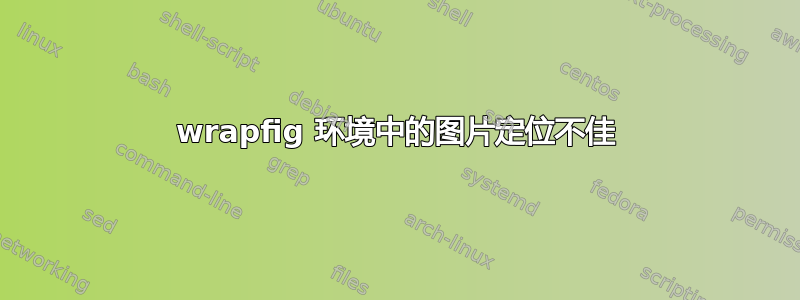
我目前正在撰写我的学士论文,对 TeX 有扎实的了解。我遇到了一个主要问题,其中一个包装图(最后一个带有 [L] 的,SDSS 望远镜图像)没有按照我想要的方式对齐。有时它在下一页或页面上的文本下方,我希望它位于那里。我也阅读了每篇帮助文章,但都没有用。我给你整章来了解整个定位。有人有什么建议可以用最少的钱解决这个问题吗?
\section{Technological Instruments \& Data}\label{tech}
The human eye is limited by the range of light it can detect. Therefore, only a small number of stars in the night sky are visible to human beings. But astronomers need to look even further out into the abyss of space to get answers to the various questions asked. The only direct way to that on earth or in the vicinity of it is via technologically advanced instruments that create an artificial eye for astronomers into space. \\
First Galilei and later other technological inventions and advancements gave astronomers one of the most powerful tools in modern astronomy: the telescope. It is the reason that astronomers can do reasonable science, although most objects in the universe are so distant. Ground-based telescopes on Earth are becoming larger and larger, ever-increasing their maximum resolution. But since the first rockets got sent into space, astronomers have sought to send telescopes into space where they could eliminate the earth's atmosphere as well as other artificial or natural contamination. Nowadays, we can look at the farthest stars in our galaxy and gain valuable information on them. Only because of this is a precise analysis of distant halo stars like in this work possible. This analysis became even more precise with the invention of spectrographs. These marvelous technical instruments give us so much more than a light-collecting telescope can: information about the composition of individual objects. By analyzing a star's measured spectra, astronomers can now get insights on parameters such as surface acceleration, temperature, metallicity, and so on. In combination, telescopes and spectrographs are the number-one weapons for astronomers. That's why most space telescopes nowadays are equipped with spectrographs to fully unfold their full potential.\\
One of the most successful space missions lately has been the \textit{Gaia} space mission, which gave astronomers on earth precise photometric and astrometric data for nearly two billion stars. The most recent data release, \textit{Gaia} DR3, is the basis for this work's analysis and will be crucial to achieving its goals. But in the future, there will still be more to come, as the European Space Agency (ESA) plans to release two more data releases. \\
As for the spectroscopy, two of the most successful surveys recently have been the LAMOST and SDSS surveys, as they provided huge amounts of spectroscopic data for many individual stars. \textit{Gaia} and LAMOST/SDSS, respectively, will deliver the basic data set upon which this works analysis builds. The three technological instruments will be discussed in the next sections.
\subsection{The \textit{Gaia} satellite}\label{Gaia}
The data used in this work has been retrieved by the \textit{Gaia} satellite (\cite{2016A&A...595A...1G}) which is a follow-up mission to the \textit{Hipparcos} satellite, both developed and launched by the European Space Agency (ESA). After its launch on December 19, 2013, the satellite (\autoref{fig:Gaia_sat}) moved to its parking spot at Lagrange Point L2, roughly 1.5 million kilometers away from earth. There, it moves on a Lissajous orbit with an orbital period of 6.3 years. Although its nominal mission should have ended on July 17, 2019, it could be extended up to the year 2025.
\begin{wrapfigure}{r}{0.5\textwidth}
\vskip-\intextsep
\includegraphics[width=0.5\textwidth]{Figures/Gaia_sat.jpg}
\caption{The \textit{Gaia} satellite (\cite{Gaia_sat})}
\label{fig:Gaia_sat}
\vskip-\intextsep
\end{wrapfigure}
The aim of the \textit{Gaia} mission was to map a significant part of the sky to create a 3D map of the Milky Way (\autoref{fig:density_map}). To achieve that, \textit{Gaia} extracted the positions, proper motions, parallaxes, and photometric data for well over one billion stars. Over the years, there have been three total data releases, the latest being \textit{Gaia} DR3 (\cite{2022arXiv220800211G}) which surveyed a total of 1.8 billion stars. Here we use this latest release, which provides us with full astrometric and photometric data for roughly 1.4 billion stars.\\
A big advantage of \textit{Gaia} DR3 is its completeness compared to \textit{Gaia} DR2 (\cite{2018A&A...616A...1G}). Not only does it contain more astrometric and photometric data, but it also provides classifications for a vast number of objects from scientific research done with \textit{Gaia} DR2.\\
A major groundwork in creating a BHB catalog with \textit{Gaia} data has been done by \cite{Culpan_2021} which is an important reference for this work. But since the analysis from \cite{Culpan_2021} was done using the early data release \textit{Gaia} EDR3, this work aims to construct a more complete catalog with the \textit{Gaia} DR3 data, building upon this previous work.
\begin{figure}[H]
\centering
\includegraphics[width=\textwidth]{Figures/The_density_of_stars_from_Gaia_s_Early_Data_Release_3.png}
\caption{Total density of stars in \textit{Gaia} EDR3 (\cite{density_map}). Brighter regions show a higher star density than dimmer regions.}
\label{fig:density_map}
\end{figure}
\subsection{LAMOST \& SDSS surveys}
Spectroscopic surveys are crucial to gaining valuable information about stars. By analyzing a star's spectra, one can, for example, determine the spectral type of most of the observed stars. Later on, it will be important to know whether the stars in our sample are at the beginning or the end of their individual lives. That is because the target stars of this work lie somewhere in between. Therefore, such surveys are crucial for us to identify different star types. Astronomers located at the Xinglong Station in the Hebei Province, China, are doing such a significant survey, which is called LAMOST (Large Sky Area Multi-Object Fibre Spectroscopic Telescope). At an altitude of 960 m stands a reflective Schmidt telescope (\autoref{fig:LAMOST_tel}) which is fine-tuned for optical wavelengths between $\lambda$=370nm and $\lambda$=900nm.
\begin{wrapfigure}{r}{0.5\textwidth}
\vskip-\intextsep
\includegraphics[width=0.5\textwidth]{Figures/LAMOST_tel.jpg}
\caption{The LAMOST telescope at the Xinglong Station, China (\cite{LAMOST_tel})}
\label{fig:LAMOST_tel}
\vskip-\intextsep
\end{wrapfigure}
LAMOST consists of two primary mirrors with different segmentation. Both mirrors are perfectly aligned with each other to correct and focus incoming light through optical fibers towards one of the sixteen 250-channel spectrographs. At the very end, the photons come to a stop at a CCD detector with 4000$\times$4000 pixels across. \\
With this setup at hand, LAMOST aimed for a spectroscopic survey of more than ten million stars in our Milky Way over a five-year span. Not only stellar spectroscopic but also extra-galactic spectroscopic data was obtained during its observations. Over the years, there have been several public data releases, the most recent being DR8 (\cite{LAMOST_dr8}) which is used for the spectroscopic analysis in this work. This set of data provides roughly 10.3 million star spectra. \\
Another survey used in this work besides LAMOST is the SDSS (Sloan Sky Digital Survey). This project is financed by the Alfred P. Sloan Foundation, which provided a 2.5 m optical
mirror telescope (\autoref{fig:sdss_tel}) stationed at the Apache Point Observatory, New Mexico, from the beginning (Sloan Foundation Telescope).\\
\begin{wrapfigure}{L}{0.5\textwidth}
\vskip-\intextsep
\includegraphics[width=0.5\textwidth]{Figures/SDSS_telescope_new.jpg}
\caption{The Sloan Foundation 2.5m Telescope at the Apache Point Observatory (\cite{SDSS_tel})}
\label{fig:sdss_tel}
\vskip-\intextsep
\end{wrapfigure}
Other than LAMOST, the Sloan Foundation Telescope collected photons with its camera in the range of $\lambda$=380nm to $\lambda$=920nm and analyzed them with thirty CCD chips consisting of 2048$\times$2048 pixels each until 2009, when the camera was decommissioned. After 2009, the telescope exclusively worked spectroscopically where the photons were transported to the spectrograph via glass fibers.\\
Following later phases of SDSS, the survey was complemented by the Iréné du Pont Telescope at the Las Campanas Observatory, Chile, located in the Southern Hemisphere, to obtain even more spectra and crucial data. \\
After more than 20 years of work, SDSS was able to create one of the most complete maps of our universe. It could especially shine light on the mysteries of the universe's expansion by analyzing stars, galaxies, and quasars in their redshifts. Furthermore, it provided important results in favor of the theory of a flat universe.
\clearpage